Proceedings of the 3rd South American International Industrial Engineering and Operations Management Conference, Asuncion, Paraguay, July 19-21, 2022
Automation of a Methanol Storage Center for Application in
Heat Treatment Furnaces
Gian Ricardo Corrêa Silva
Federal Institute of São Paulo (IFSP) – Sorocaba Campus
Sorocaba, Sao Paulo, Brazil [email protected]
Vitor Mendes Caldana [email protected]
Sergio Shimura [email protected]
Abstract
Hazards inherent to chemical products must be minimized to ensure the health of workers in processes where flammable and toxic liquids are stored, the integrity of piping, valves and equipment throughout the system must be ensured until its application. The main aspects to be considered for the storage of chemical products are compatibility between materials, temperature, ignition control, ventilation, segregation and identification. However, many potential hazards are associated with the storage and handling of hazardous chemicals. The purpose of this work is to present a technical solution for the automation of the mechanical handling operation performed by the operator, the filling of the tanks, level control, cleaning (purge), pressurization of the system with inert gas, reversal and rotation of the tanks, in order to guarantee the supply of liquid methanol with controlled pressure and flow rates used in atmospheres of heat treatment furnaces and reduction of metallic powders, eliminating operator handling, increasing the safety of the system, and making the process more robust. The methodology used for this purpose starts with an analysis and algorithm of the electrical components for automation, PLC programming, operation simulation via Festo's CodeSys software, and IoT techniques aimed at industry 4.0. This work presents a simplified technical solution for the automation of a methanol supply center, in order to prevent the operator from handling the toxics and highly dangerous chemicals products that are applied in atmospheres of heat treatment furnaces.
Keywords
Storage; retrofit; safety; atmosphere; furnace; heat treatment
1.Introduction
Synthetic gaseous atmospheres based on nitrogen and methanol are used in furnaces for the reduction of metallic powders and during the heat treatment of metals (Hryha et al. 2012; Silva et al. 2012). Among many processes that use dissociated methanol atmospheres, we can mention the reduction of metallic powders (copper, for example), and the carburizing and hardening treatments in automotive parts (Plicht et al. 2002). In these applications, methanol is the main source of reducing gases, where the methanol molecule is dissociated (“cracking”) at high temperatures (i.e., range from 800°C to 1000°C) generating gases such as hydrogen and carbon monoxide (Abbasi et al. 2015), as shown in figures 1 and 2.
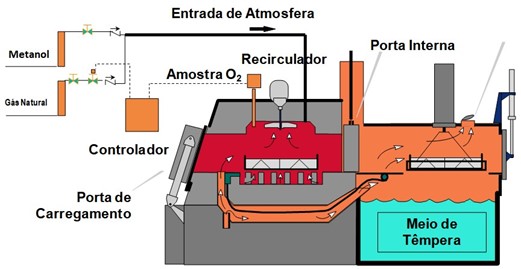
Figure 1: Schematic of an application of liquid methanol to form the atmosphere in an integrated hardening furnace.
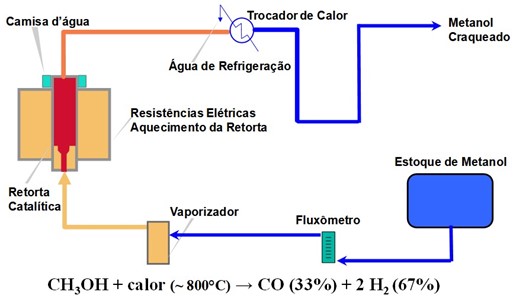
Figure 2. Schematic of a generator of reducing gases (hydrogen and carbon monoxide) from the dissociation of methanol at high temperatures.
Normally, different mixing ratios apply for each type of steel and heat treatment, which is based on nitrogen gas, alcohol (i.e., methanol or ethanol or isopropyl alcohol), and a hydrocarbon gas for carbon enrichment and correction of the chemical potential of the atmosphere (i.e., propane or liquefied petroleum gas or natural gas or acetylene) (Mullinger and Jenkins 2008).
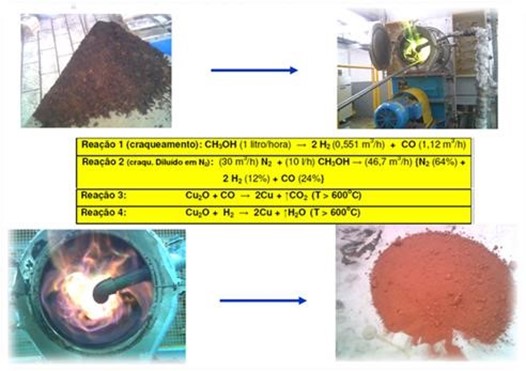
Figure 3. Application of methanol in the furnace to obtain a reducing atmosphere during the thermal processing to manufacture of metallic powders (ex., copper powder).
As shown in Figure 3, during thermal processing, special use and handling of flammable gases and liquids is necessary to minimize possible harmful reactions. Fire and explosion risks can be controlled by operating procedures that prevent the formation of fuel-oxidant mixtures, and also by eliminating ignition sources such as sparks, flames or other heat sources (Mullinger and Jenkins 2008; NR-20 2019).
However, flammable mixtures burn when ignited and may explode under certain conditions. Some flammable gases may accumulate as pockets in enclosed areas, and explosion may occur at temperatures above auto-ignition temperature or by other heat sources if the system is not properly cleaned with inert gas (i.e., purged with nitrogen) in order to condition the chambers inside furnaces and reactors at concentration levels below 1% oxygen (NFPA 30 2021; NFPA 86 2021).
In addition, it is considered that safe installations and good practices of a methanol storage and supply system must comply with technical specifications and Brazilian standards, such as NR-10, NR-13, NR-16 and NR-20, and also with international standards such as NFPA 30 (National Fire Protection Association), NFPA 70, NFPA 86 and OSHA (Occupational Safety and Health Administration).
This automation project will control by timer or by the weight of the methanol tanks, the opening and closing of solenoid valves, the pneumatic pumping of methanol, supplying the piping network and the flow control panels, while the other tank is in the waits until it reaches the predetermined minimum level of operational safety for reversal, where new cycles are started successively.
2.Literature Review
2.1 The System of Storage and Supply of Methanol
As a source of methanol supply for applications in metal processing atmospheres, liquid alcohol is stored in carbon steel tanks being pressurized by nitrogen to supply the piping line, a flow control panel to distribution and injection into the chambers of the furnaces (NR-13 2019; Yao et al. 2017; Yao et al. 2018). Figure 4 shows details of a methanol storage and supply center.
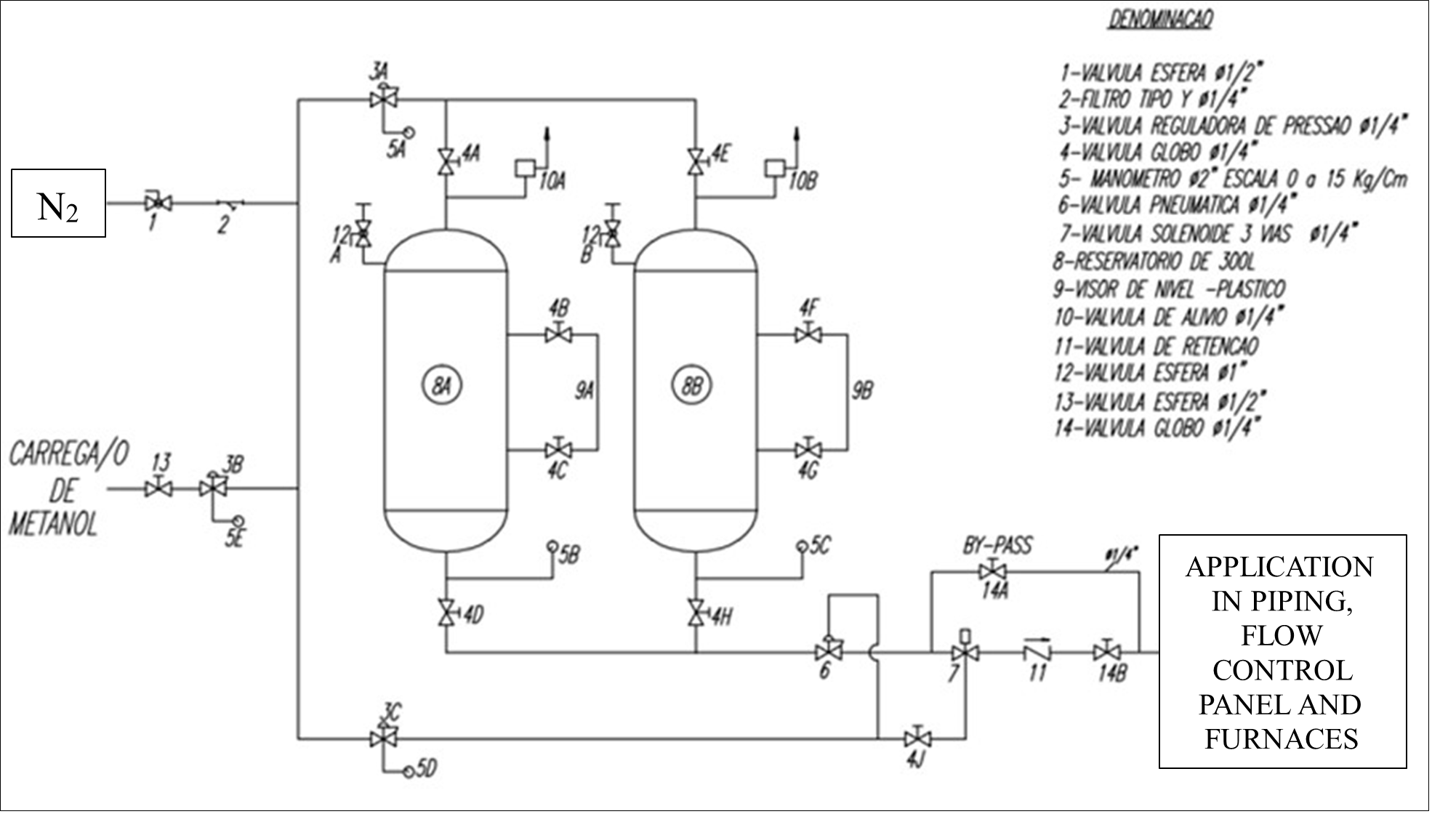
Figure 4. Mechanical design of a nitrogen-pressurized methanol storage and supply center. Source: Author's compilation.
2.2 Safety Considerations and Operational Risk
For an employee of a chemical or metallurgical industry, the operational process of handling methanol, including the steps of purging (cleaning), checking the level, filling, pressurizing and reversing the tanks that serve the applications is very dangerous, as there are risks of methanol leakage that can cause fire and explosion, risk of contamination of the stored product, risk of inhalation of high concentrations in the air that can cause irritation of the mucous membrane, headaches, drowsiness, nausea, confusion, loss of consciousness, digestive disturbances and even death (NR-16 2019; NR-20 2019; FISQP 2021).
Depending on the severity of the poisoning and the promptness of treatment, survivors may recover completely or may experience permanent blindness, vision disturbances and/or nervous system effects. Airborne concentrations in excess of 1000 ppm (i.e. 0.1% by volume) can cause irritation of membranes and mucous. Skin contacts and ingestion of small amounts of methanol can cause blindness or death (MSDS 2005; MSDS 2021).
2.3 Justification
To avoid problems and discussions in labor claims that may result in future compensation to the worker, due to the dangerousness of the operation and exposure to methanol for years. Hazards inherent to chemical products must be minimized to ensure the health of workers in processes where flammable and toxic liquids are stored.
With the advances, requirements and current methodologies in the implementation of strategic initiatives of Industry 4.0, this automation project proposes to develop a viable solution for the operation of a sustainable methanol storage and supply center, ensuring innovation and technological development, connectivity and integration between devices, machines and equipment, used in the application of methanol in furnace atmospheres for processing metal thermal. Therefore, dispense with operator handling, increase system security, and make the process more robust (Canas 2021; Enyoghasi 202; Javaid 2021).
The methodology used in this project will start with an analysis of the operation and the algorithm of the electrical components necessary for this automation, the PLC programming, the creation of a human-machine interface (HMI), the simulation and validation of the automated operation via Festo's CodeSys software. Moreover, with the application of IoT techniques aimed at Industry 4.0.
2.4 Objectives
The proposed solution aims at the automation of the methanol plant, including the provision of an electrical panel for application and control of the methanol level, pressurization and depressurization of the system with nitrogen, purge with inert gas for cleaning and safety, automatic reversal of steel tanks that connect to the piping network that supply the heat treatment furnaces, eliminating the need for operator management inside the plant.
In this way, the automated system will monitor via timer or monitor the weight of the methanol tanks, pressurization and depressurization of the tanks, opening and closing valves, we will always have a tank in use and another full (reserve) waiting, when it is verified that the level of tank in use has reached the predetermined level for replacement, new cycles are started repeatedly.
3.Methods
Assembly of a mechanical panel with solenoid valves, manual ball valves and valves with pneumatic actuators, to carry out the automatic reversal (change) of the methanol tanks. Use of 02 (two) load cells for weighing the tanks, which will be interconnected with the panel for checking the tank level and automatic change. The system will have an electrical panel with a programmable logic controller (PLC), which will control the methanol level and the release for filling the two reservoirs. In this case, a human-machine interface (HMI) screen is provided on the front of the panel that will allow the operator to check information on the levels and control of the valves.
The system will automatically change the tanks according to the pre-set values in the human-machine interface (HMI), if it is necessary to actuate the valves manually, the operator can activate the same valves by means of a command on the HMI screen. In addition, a pressure regulator for the nitrogen line will be inserted, which will pressurize the tanks and activate the valves as pneumatic actuators. The plant has 02 (two) methanol tanks with a capacity of 1000 to 2000 liters, in carbon steel and resistant to 20 kgf/cm², including mechanical and safety handling valves, pressure gauges and level sights, as showed as figure 5 (b). Figure 5 gives an overview of the primary storage place for methanol (a), and nitrogen pressurized methanol supply plant (b).
It will use a power supply for the electrical panel, and safety signs in accordance with current regulations. Standard materials of connections, valves and pipes in steel or annealed copper, 15mm in diameter, first class, considering all welded joints by brazing with appropriate addition material in alloy (silver-copper).
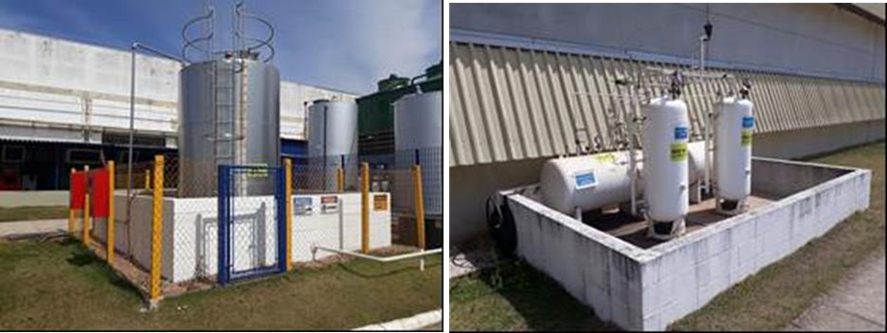
< >(b) Figure 5: The images above show (a) the general methanol storage area, and (b) where the automation project will be implemented.
3.1 Specification of Electrical Components
In this automation project, some electrical equipment was selected as shown in Figure 6. In sequence, we can observe a programmable logic controller (PLC), a screen for human-machine interface (HMI), solenoid valves normal open or closed, and a force transducer as a load sensor.
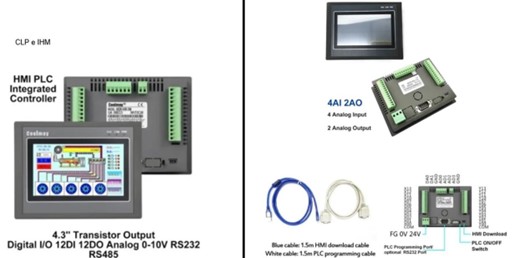
(a)
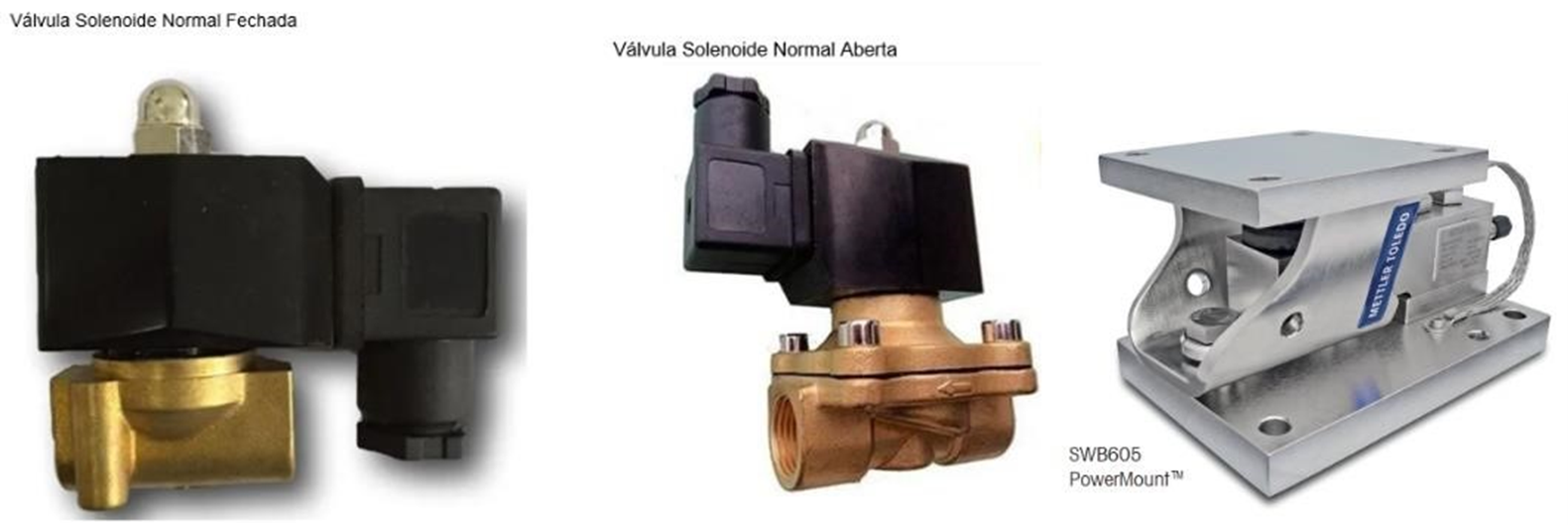
(b) (c) (d) Figure 6: Images of the electrical components for the automation of the methanol storage and supply system.
Indicating (a) PLC; (b) Normal Open Solenoid Valve; (c) Normal Closed Solenoid Valve; and (d) Load Sensor (force transducer).
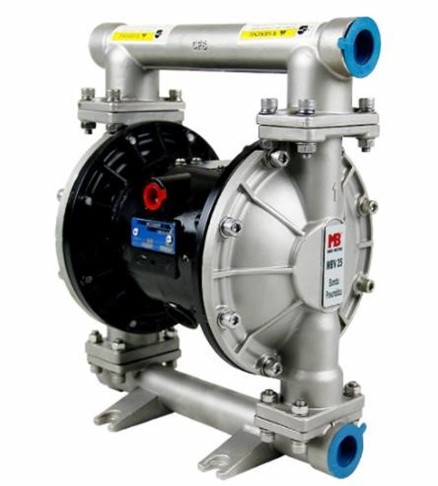
Figure 7: Images of the Actuator or Pneumatic Pump.
3.1.1 Electromechanical Actuators (Normal Closed and Normally Open Solenoids) Solenoid valve is an electromechanical device used to control the flow of liquid or gas. The solenoid valve is controlled by electric current, which passes through a coil. When the coil is energized, a magnetic field is created, causing a plunger inside the coil to move. Depending on the valve design, the plunger will either open the solenoid valve or close the valve. When electrical current is removed from the coil, the valve will return to its normal (no power) state. In direct acting solenoid valves, the piston directly opens and closes an orifice inside the valve.
3.1.2 Force Transducer (Load Cell) The force transducer measures the tension and compression forces acting on the sensor. The load cell is an example of a force transducer, as they are specially designed for use in weighing equipment (figure 6-d).
3.1.3 Actuator or Pneumatic Pump A Pneumatic Actuator (see figure 7) is a device that converts the incoming pneumatic energy into kinetic energy, that is, into mechanical movement. The types of Pneumatic Actuators can be single acting or double acting and even linear or rotary.
4.Results and Discussion
4.1 Positioning of Sensors and Actuators Installed in the Storage System
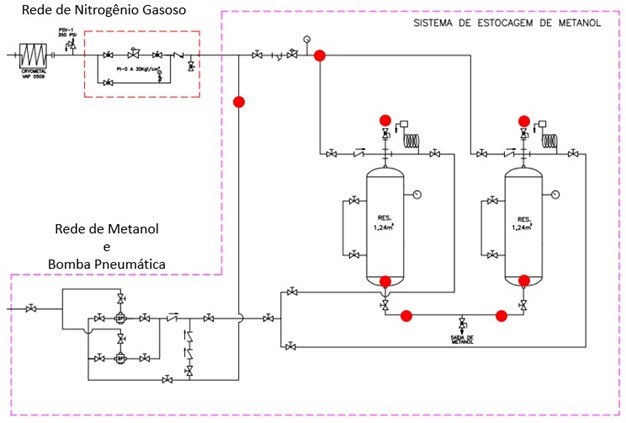
Figure 8: The image shows the position of the electrical devices in the methanol plant.
Table 1 lists the electrical components that are used for the automation of the nitrogen-pressurized methanol storage and supply system (see figure 8), and which were used for the analysis of the operation and process algorithm. Including the main functions of electrical devices and the description of the signal, being normal open (NO) and normal closed (NC).
Table 1: List of electrical components selected for central automation.
DEVICE |
SIGN DESCRIPTION |
ROLE |
S1 |
SOLENOID – Normal Open |
Energize Panel |
H0VM |
RED SIGNALLER |
Light bulb |
CQ1 |
LOAD CELL* |
Level control (*optional) |
CQ2 |
LOAD CELL* |
Level control (*optional) |
S2 |
SOLENOID – Normal Open |
Button for vent opening and depressurization |
S3 |
SOLENOID – Normal Open |
Button for vent opening and depressurization |
H0AM |
YELLOW SIGNALLER |
Light bulb |
S4 |
SOLENOID – Normal Closed |
Supply Button via Tank 01 |
S5 |
SOLENOID – Normal Open |
Supply Button via Tank 02 |
S6 |
SOLENOID – Normal Open |
Activates/deactivates the pneumatic methanol supply pump |
S7 |
SOLENOID – Normal Open |
Methanol release button for consumption |
H0VD |
GREEN SIGNALLER |
Light bulb |
BP |
PNEUMATIC ACTUATOR |
Supply Pump |
4.2 Flow of Operation of Electrical Devices in the Automated System with PLC Timers
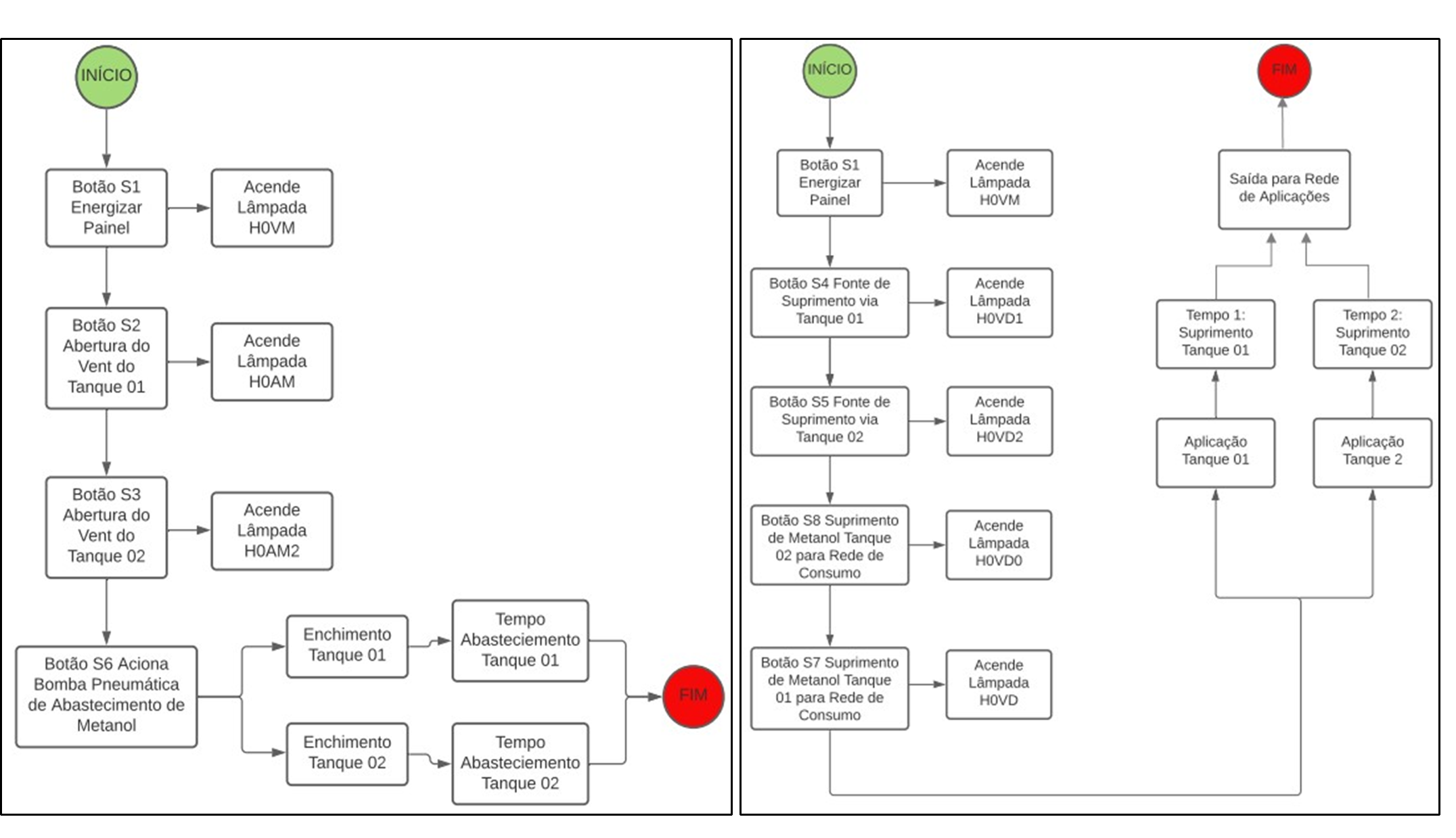
Figure 9: Automated System Operation Algorithm via PLC timers.
The figure 9 show the automated system operation algorithm via PLC timers. The images below demonstrate Festo's
CodeSys software during simulation and supply into the pipeline through the tank (TQ01), while filling the tank (TQ2), including pressure relief (Vent TQ2), nitrogen purge (N2) and the operation of the pneumatic pump (and vice versa), including the device mesh and the HMI screen (see figure 10).
|
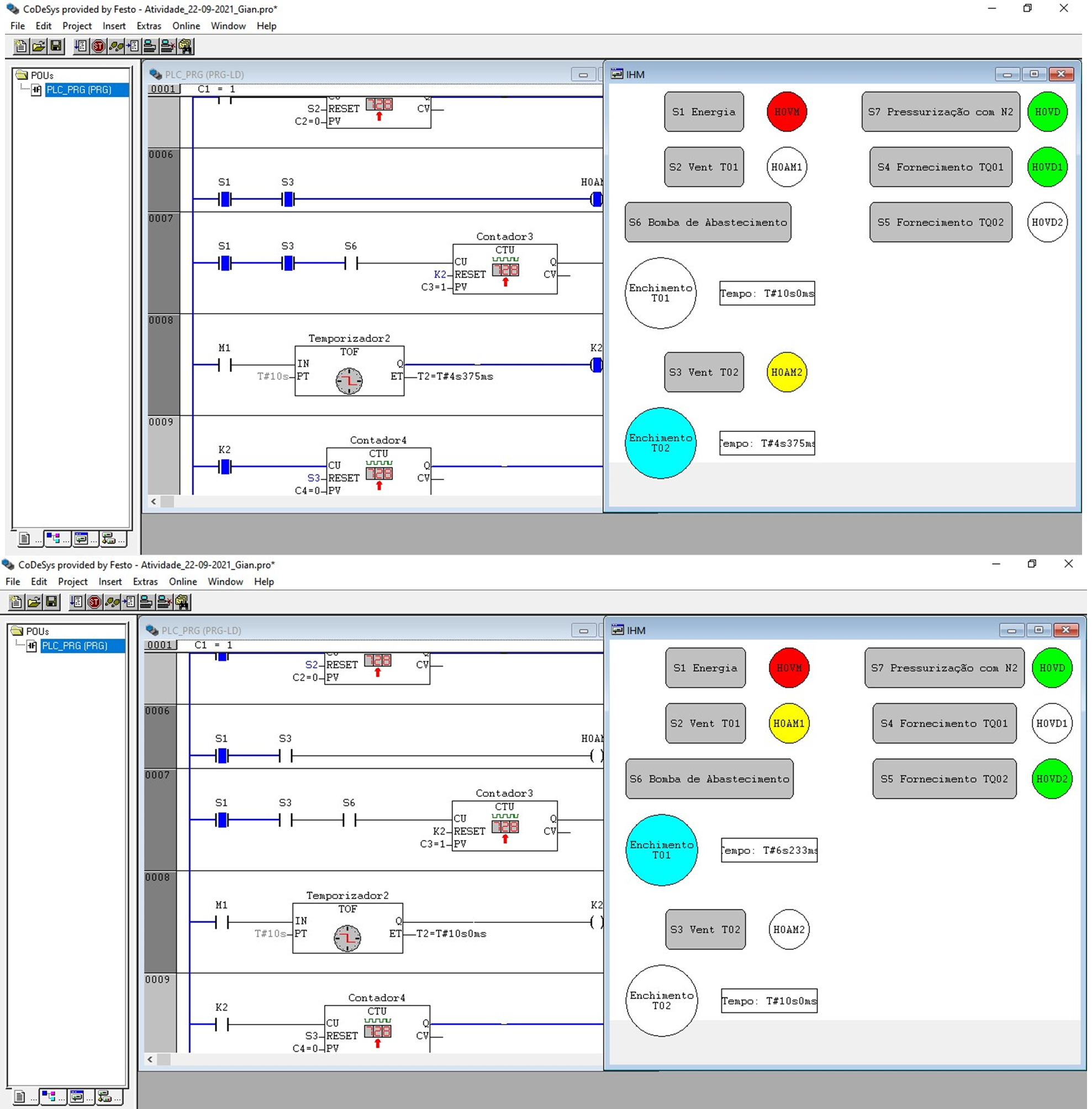
Figure 10: Device circuit loop simulation and automated system HMI screen.
4.3 Graphic Screens of the Man-Machine Interface of the Codesys Software
The figure 11, 12 and 13 represent the human-machine-interface screens showed the operations of tanks filling (TQ01 and TQ-02) alternately during methanol application.
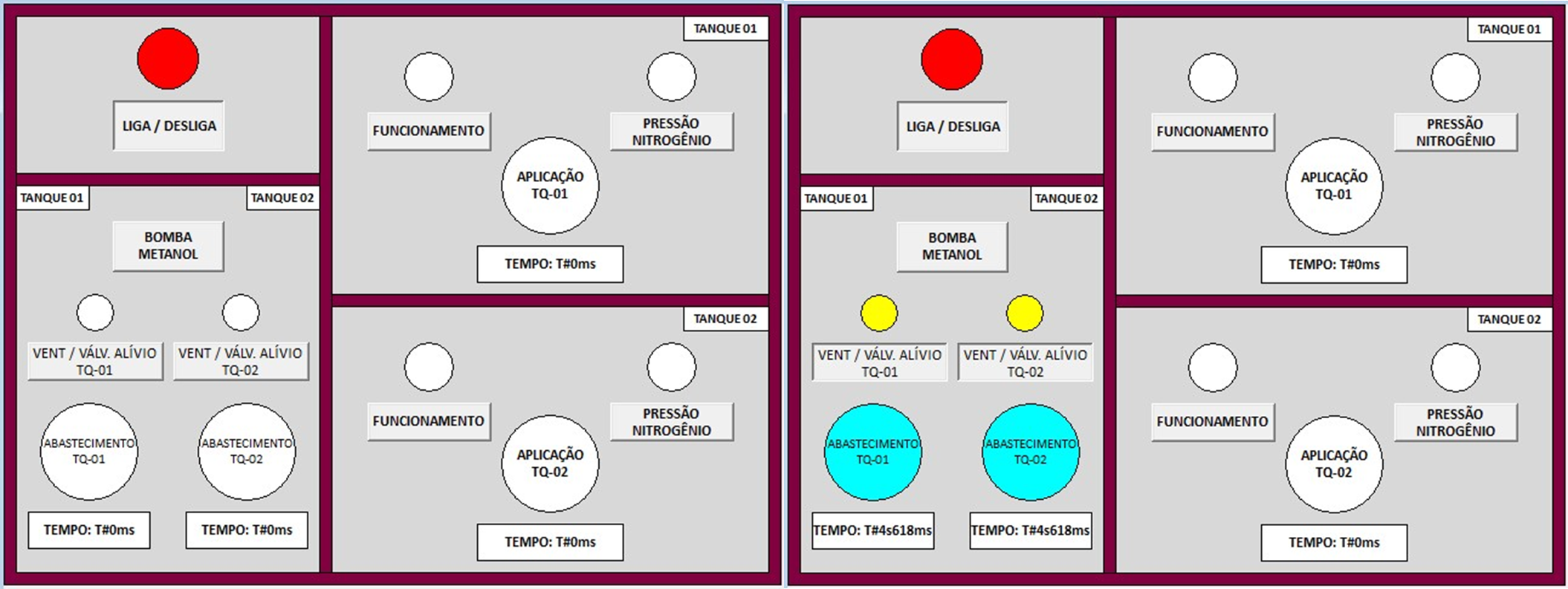
Figure 11: The right image shows the HMI panel being activated and the HMI panel during tank filling (left).
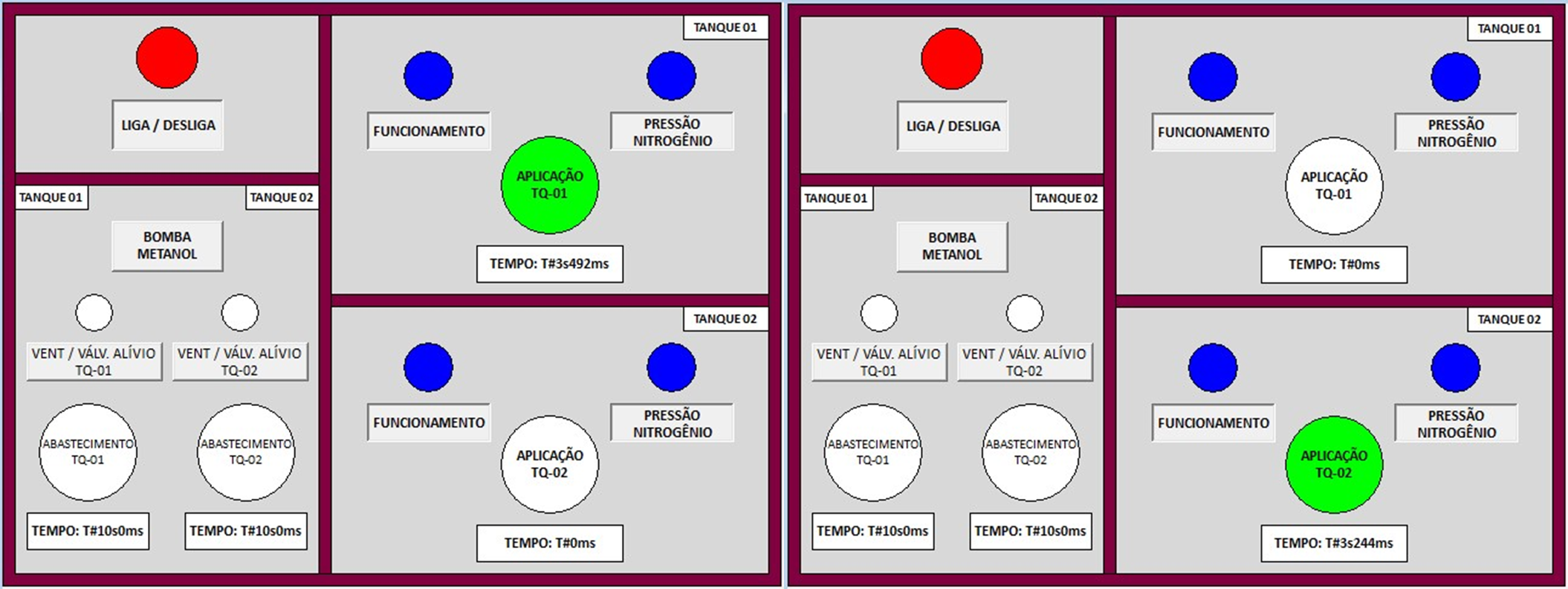
Figure 12: The right image shows the HMI panel during supply of the TQ-01, and the during supply of the TQ-02.
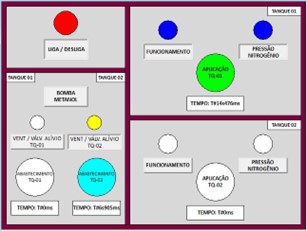
Figure 11: The image represents the HMI panel during simultaneous operations, for the supply of the TQ-01 tank and the supply of the TQ-02 tank, respectively.
4.4 Proposed Improvements
The flow of operation of electrical devices in the automated system using load cells were showed in the figure 14, it represents an analysis using the algorithm of the automated operation for the simulation via the FESTO CodeSys program (software).
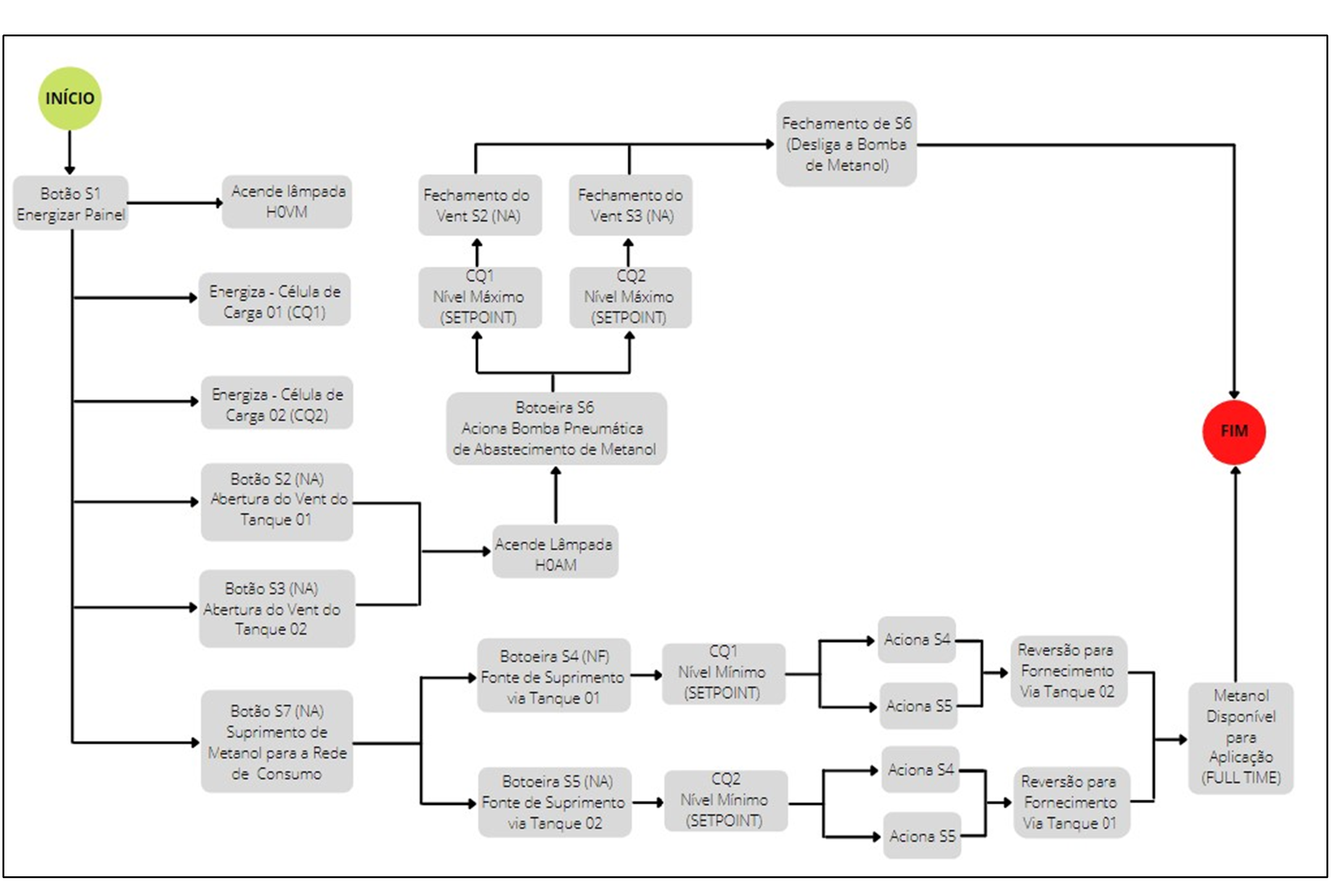
Figure 12: Automated System Operation Algorithm via load cell.
5.Conclusion
This work presented a simplified technical solution for the automation of a methanol supply center, in order to prevent the operator from handling the toxic and highly dangerous product. Festo's CodeSys software proved to be an excellent simulation tool for the proposed open-mesh and closed-mesh circuit, and validated the electrical devices inserted in the mechanical automation project of the methanol supply system. The solution proposed an automatic control for the supply of the 02 reservoirs (two tanks) of the methanol storage system, indicates the maximum and minimum level of product, promotes filling, pressurization and depressurization with nitrogen for cleaning and safety, promotes automatic reversal keeping the supply of liquid methanol stable, with constant pressure and with the flow rates used during the application in furnaces atmospheres, avoiding unwanted stops due to product shortages. For future work, we can suggest the intercommunication of the PLC and the IoT devices specified in the project, including the selection of devices, layer types, networks for wi-fi and cable signals, and types of communication protocols to be used to remote control and management of the operation.
References
Abbasi, M., et al., A Feasibility Study for Synthesis Gas Production by Considering Carbon Dioxide Capturing in an Industrial-Scale Methanol Synthesis Plant, Arabian Journal for Science And Engineering, vol. 40, no. 5, pp. 12551268, 2015.
Canas, H., et al., Implementing Industry 4.0 principles, Computers & Industrial Engineering, vol. 158, 2021.
Enyoghasi, C., and Badurdeen, F., Industry 4.0 for sustainable manufacturing: Opportunities at the product, process, and system levels, Resources, Conservation & Recycling, vol. 166, 2021.
Hryha, E., et al., On-line control of processing atmospheres for proper sintering of oxidation-sensitive PM steels, Journal of Materials Processing Technology, vol. 212, pp. 977-987, 2012.
Javaid, M., et al., Significance of sensors for industry 4.0: Roles, capabilities, and applications. Sensors International. vol. 2, 2021.
MSDS Material Safety Data Sheet – Methanol, Kel-Ex Agencies Ltd., P.O. Box 52201, Lynnmour, RPO, North Vancouver, B.C. V7J 3V5, Methanex Corporation, 2005.
MSDS Material Safety Data Sheet – Methanol, Air Products and Chemicals, Inc., 7201 Hamilton Blvd., Allentown, PA 18195-1501, Available: www.airproducts.com/msds, Dec 12, 2021.
Mullinger, P and Jenkins, B., Furnace control and safety, Industrial and Process Furnaces Principles, Design and Operation, pp. 287-333, 2008.
NR-10 Safety in Electricity Lectricity Facilities and Services. Ordinance SEPRT 915, of 07/30/2019,
Available: https://www.gov.br/trabalho-e-previdencia/pt-br/composicao/orgaos-especificos/secretaria-de- trabalho/inspecao/seguranca-e-saude-no-trabalho/normas- regulatory agencies/nr-10.pdf, Dec 12, 2021.
NR-13 Boilers, Pressure Vessels, Pipes and Metallic Storage Tanks. Ordinance SEPRT 915, of 07/30/2019, Available:
https://www.gov.br/trabalho-e-previdencia/pt-br/composicao/orgaos-especificos/secretaria-de- trabalho/inspecao/seguranca-e-saude-no-trabalho/normas- regulatory agencies/nr-13.pdf, Dec 12, 2021. NR-16 Dangerous Activities and Operations. SEPRT Ordinance No. 1,357, of December 9, 2019. Available:
https://www.gov.br/trabalho-e-previdencia/pt-br/composicao/orgaos-especificos/secretaria-de-trabalho/ inspection/safety-and-health-at-work/regulatory-norms/nr-16-updated-2019.pdf, Dec 14, 2021.
NR-20: Safety and Health at Work with Flammable and Fuel. SEPRT Ordinance No. 1,360, of December 9, 2019. Available: https://www.gov.br/trabalho-e-previdencia/pt-br/composicao/orgaos-especificos/secretaria-de- trabalho/ inspection/safety-and-health-at-work/regulatory-standards/nr-20-updated-2021-with-prc.pdf, Dec 13, 2021.
NFPA 30 Flammable and Combustible Liquids Code. Available: https://www.nfpa.org/codes-and-standards/all- codes-and-standards/list-of-codes-and-standards/detail?code=30, Dec 12, 2021.
NFPA 86 Standard for Ovens and Furnaces. Available: https://www.nfpa.org/codes-and-standards/all-codes-and- standards/list-of-codes-and-standards/detail?code=86, Dec 12, 2021.
Plicht, G., Frey, J., et al. Accelerated and improved carburizing with MFC controlled nitrogen-methanol atmospheres, Surface Engineering, vol.18, pp. 171-173, 2013.
Silva, G.R.C., et al., Techniques to Optimize, Control and Stabilize the Atmosphere in a Sintering Furnace. Materials Science Forum, vol. 727-728, pp. 404-411, 2012.
Yao, C., Hu, J., Geng, P., et al, Effects of injection pressure on ignition and combustion characteristics of diesel in a premixed methanol/air mixture atmosphere in a constant volume combustion chamber, Fuel, vol. 206, pp. 593602, 2017.
Yao, C., Hu, J., Geng, P., et al, Visualization of combustion characteristic of diesel in premixed methanol–air mixture atmosphere of different ambient temperature in a constant volume chamber, Fuel, vol. 174, pp. 242-250, 2016.
Biographies
Gian Ricardo Corrêa Silva holds a master's degree in Science and Engineering of Materials and Metallurgy from the University of São Paulo in the Inter-Units Program (USP - 2005), a degree in Materials Engineering from the Federal University of São Carlos (UFSCar - 2001), and Specialist in Technologies for Industry 4.0 by the Federal Institute of São Paulo (IFSP - 2022). He has over 20 years of experience in the metallurgical industry, equipment and commercial technologies. He is currently a teacher of higher education at FATEC and industrial technical consultant.
Vitor Mendes Caldana began the academic career with a technician course in Electronics from Liceu de Artes e
Ofícios (1999) followed by an undergraduate degree in Electronic Engineering from Universidade Presbiteriana
Mackenzie in 2004. In 2016, finished his Master´s course in Industrial Engineering with Quality of Engineering Education and its Relation to Regional Development as his area of research. In 2014 began his teaching career in FIEB as a substitute teacher and after as an associate professor for the Technical Course of Electronics. In 2016, whilst holding the position of Service and Projects Manager at Caltronic, left the company for full-time dedication in teaching in IFSP. In 2018 began his Research Group in Industry 4.0 and between 2019 and 2020, along with his colleagues, designed and implemented the first Post-Graduate Program in Industry 4.0 of IFSP. He is currently beginning his PhD research in the fields of IoT and Industry 4.0.
Sergio Shimura holds a degree in Electrical Engineering from the University of São Paulo (1987), a Master's degree in Electrical Engineering from the University of São Paulo (1992); PhD in Electrical Engineering from the University of São Paulo (1999); post-doctorate in reliability of photovoltaic generation systems (2016); Certified Reliability Professional (2012). EBTT Professor at the Federal Institute of São Paulo since 2016.
© IEOM Society International